You Versus The Atmosphere (100% FiO2?)
- Sam Ireland
- Jan 14, 2021
- 10 min read
When it comes to delivering oxygen, we are in a constant battle with the atmosphere around us. When the oxygen content of the ambient air is not currently enough to sustain our patient's oxygenation, what do we do? Sometimes, we think we're putting the patient on nothing but pure oxygen.
“We now have the patient on 100% oxygen, but their saturation is not improving.”
How often do we think that a patient is receiving 100% oxygen? I remember I was taught that a non-rebreather mask would deliver 100% oxygen, and so would a bag valve mask device. Is this true? Can we say a device delivers a certain amount of FiO2? Before we dive into that question, let’s quickly define FiO2 and understand what influences it.
FiO2
FiO2 is the fraction of inspired oxygen. Let’s break that down.
A fraction is a part of a whole number
Inspired means we’re breathing it in
O2 is obviously for oxygen

So, FiO2 is what percent of the air we breath in that's made up of oxygen. That’s pretty simple, right? In our atmosphere we have 20.95% oxygen (we always round up to 21%), so what’s our normal FiO2? It’s about 21%. However, our atmosphere is a much more simple concept than our oxygen delivery devices. Why? The atmosphere is always there - it's endless. The atmosphere doesn't have a flow rate, and it isn't mixing with other concentrations of gas. The atmosphere is simply all around us. What about oxygen delivery devices? Can we say that they deliver a set amount of FiO2?
Let's compare and contrast two examples and see if we can make an absolute statement about an oxygen delivery device. For both examples, we'll use a nasal cannula flowing at 2 Lpm. According to tables that tell you how much FiO2 you should get from a nasal cannula, we should get 29% FiO2 from a nasal cannula flowing at 2 Lpm (~4% above 21% for each Lpm).
Example one:
5' 2" tall female with minimal shortness of breath. She is breathing in at a pretty normal rate and volume. What percent of the air she takes in is made up of oxygen?
Let's assume she's breathing in with an inspiratory flow rate of 20 L / min. The formula would go like this:
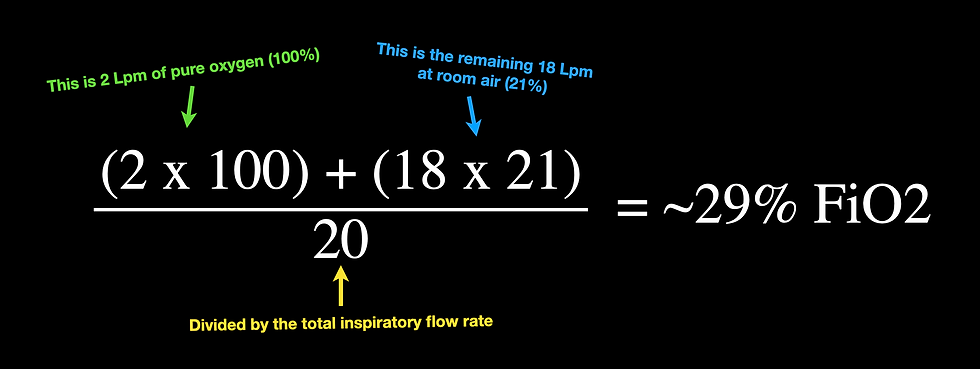
Pretty accurate! Now let's try this with another patient who is inhaling more quickly.
Example two:
6' 5" tall male with severe shortness of breath. He is breathing at a very quick rate and is in taking rather large tidal volumes. What percent of the air he takes in is made up of oxygen?
Let's assume he's breathing in with an inspiratory flow rate of 50 L / min. The formula would go like this:
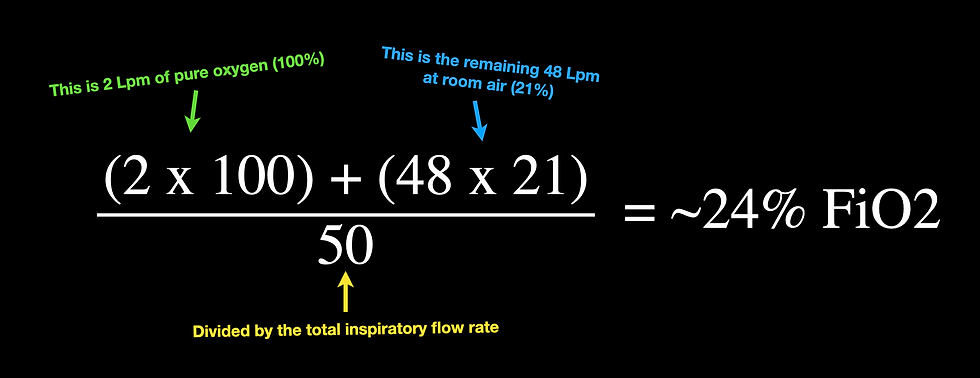
Here we can see that the FiO2 delivered by a device is completely relative to how quickly the patient is breathing in. The bigger the patient and the faster they inhale, the lower the FiO2 gets. Inspiratory flow rates usually sit around 20 Lpm, but in respiratory distress, this inflow could go as high as 60, 70 even >100 Lpm. For reference, 60 Lpm would be the equivalent of us bringing in a 500mL tidal volume over about half of a second ((500 x 60) / 0.5 = 60,000 or 60 Lpm) (formulas are taken from Cairo, 2014). Just as an extra example of a NRB mask at 15 Lpm and a patient inhaling at a pretty quick 80 Lpm, the patient would only be getting ~36% FiO2. That's a pretty far cry from the 100% I was told it would deliver.
Quick pause - which gas law are we talking about here?
...
.....
Dalton's law! What's that one about again? Dalton's law states that in a mixture of gases, the total pressure exerted is equal to the sum of the partial pressures of the individual gases. What does that mean for our purposes today? It means that the pressure of the oxygen from our gas source and the oxygen from the atmosphere make up the total pressure of the oxygen being inhaled.
Alright, let's get back to FiO2 and flow.
The above example is in contrast to what is usually stated when it comes to the FiO2 that a nasal cannula can deliver. Normally when we see FiO2 delivery tables for the nasal cannula it looks like this (taken from MDCalc):
~4% FiO₂ added above room air per 1 L/min
• Room air = 21%
• 1 L/min = 25%
• 2 L/min = 29%
• 3 L/min = 33%
• 4 L/min = 37%
• 5 L/min = 41%
• 6 L/min = 45%
However, like most things, it's all relative. If I took a long, slow breath in through my nose at 6 Lpm, and my nasal cannula is flowing at 6 Lpm, I would get 100% FiO2.
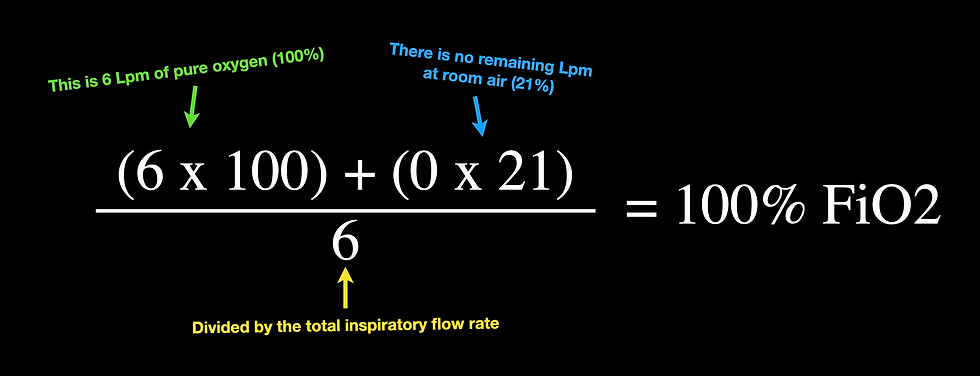
Here we can clearly see that the closer our inspiratory flow rate gets to the flow rate of the device we're using, the closer we get to 100%. This has been investigated by Markovitz et al (2010) as well, finding that the increase in FiO2 from a device is lower than usually expected due to inspiratory flow rates being much higher than the devices provide.
How ya doin' so far? That's a lot of boring math. However, it gives us some evidence as to why we do and see certain things. Remember how we've always been taught to coach a patient to take those long, slow breaths to control their breathing? This is one reason why we do that. The slower and deeper they breathe, the more effective our oxygen delivery device is. This is also why we see certain oxygen delivery devices work better for some patients than others. We are also starting to see that references for FiO2 delivered by various devices are approximate (and become less accurate with worsening dyspnea). By the way, here are some other common devices and their FiO2 ratings according to Kacmarek et al (2017).
Simple Face Mask flowing at 5-10 Lpm = 35-50% FiO2
Partial Non-rebreather mask (what we all carry) flowing at a minimum of 10 Lpm = 40-70% FiO2
High-flow nasal cannula flowing at up to 60 Lpm = 35-90% FiO2 (titratable)
Oxyhood flowing at >7 Lpm = 21-70% FiO2
Isolette flowing at 8-15 Lpm = 40-50% FiO2
Again, these FiO2 ratings are approximate and will fall with increasing inspiratory flow rates. You can reference charts like this to get a general idea of oxygen percent delivered, but we have to keep in mind that the patient that's sucking air like Kirby is going to suck in a bunch of room air and dilute that FiO2.
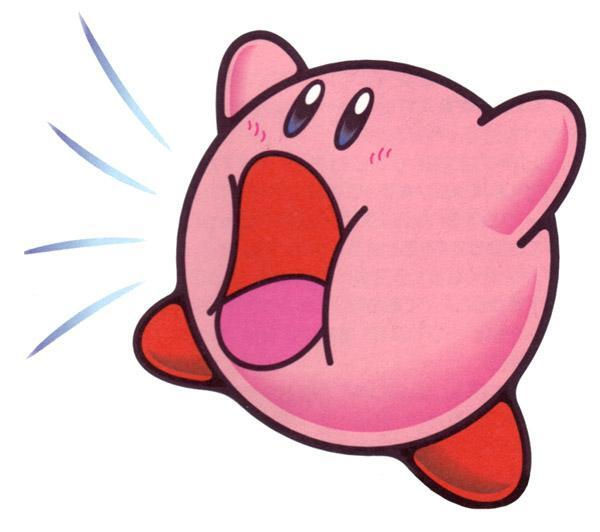
You Probably Don't Even Carry High Flow Devices
"We put the patient on high-flow." Not likely. Why not? We've long been told that a "high-flow" device is something like our non-rebreather mask, a simple mask, or maybe even our disposable CPAP device. However, what are you setting your flow rates to? 15 Lpm? Again, judging a device as "high-flow" is all relative. So, what is a "high-flow" device?
A high-flow device is one that meets or exceeds the inspiratory flow demand of the patient.
Could we consider a non-rebreather to be a high-flow device? Only if the patient is breathing at 15 Lpm or less. What if we added a nasal cannula under the mask at an additional 15 Lpm? Then, we could say this combination is high-flow only if the patent is breathing at 30 Lpm or less. What if our CPAP is running at 20 Lpm? Again, we could only say this is a high-flow device if the patient is breathing at 20 Lpm or less. This means that if we are truly to deliver high-flow oxygen (especially in a patient with dyspnea) we would likely need a BiPAP machine, a ventilator, or a high-flow nasal cannula device such as the Airvo HFNC. (The non-rebreather, simple mask, or cannula from above could be considered high-flow if their flows were increased drastically to overcoming the inspiratory flow rate of the patient.) These devices (the real high flow devices) use either mechanical support to increase their flow rate or use very high flow rates from a supply that ensures the FiO2 of the patient is completely controlled. As long as there is room air being entrained (brought in from anywhere besides the device), the device cannot be said to be a high-flow device (Chang, 2014).
Why isn't this a high flow device? Because it entrains room air.

Why is this a high flow device? Because it is not entraining any room air.
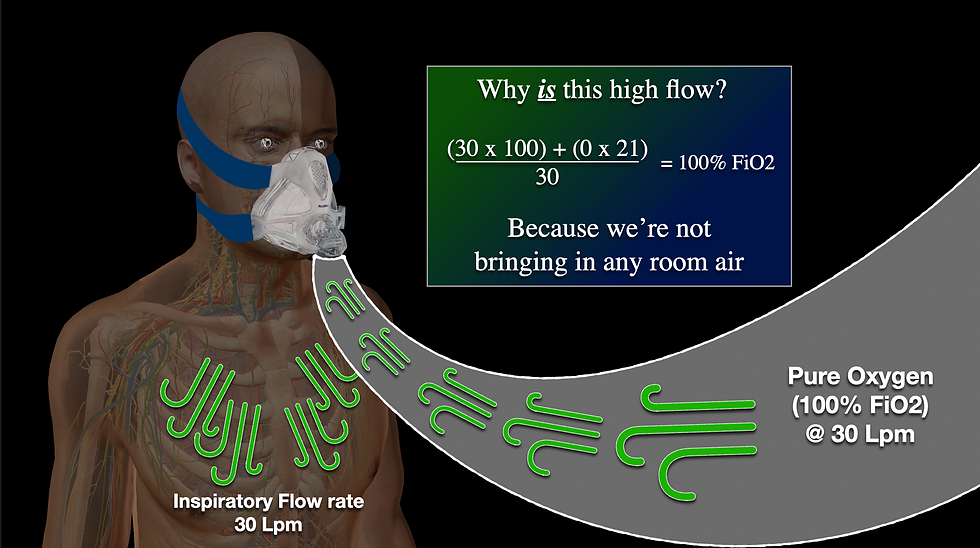
Before we move too far away from this topic of flow, I want to quickly mention something about devices that can restrict flow. I realize that this has been beaten to death by a very common picture used by Dr. Richard Levitan that shows a hypoxic patient pulling their non-rebreather mask off of their face. But, by the off-chance that you haven't heard of this concept, I'll briefly mention it here. So, why would a patient want to rip a mask off of their face?
Simply put, supply and demand. If the mask restricts flow by not allowing the patient to entrain enough flow (extra flow outside of the oxygen delivery), they will feel like they're suffocating. This is one reason why patients don't like simple and non-rebreather masks, but generally tolerate the OxyMask - the larger holes in the OxyMask never restrict flow, unlike the small holes found on the other masks.
Wrapping this point up... If the patient is pulling the mask off, they probably need more flow. Try sprinting up a hill and then breathing through a coffee straw - you'll realize flow restriction when you need air now will induce a special kind of panic.
Does high-flow always mean 100% FiO2?
No. High-flow means that we have complete control over the FiO2 that the patient is getting. When we place a patient on a ventilator, we might adjust the FiO2 to 50%. This does not mean that the device is not high-flow, it simply means we are ensuring that no matter how fast the patient breaths in, they are getting 50% (because that's what we want to deliver). If you really had to bring it down to a basic level, you could say that high-flow means control - the patient cannot out-breathe the device to get an FiO2 different than what you are providing. The HFNC such as an Airvo mentioned above is a good example of this. HFNC devices mix ambient air (medical air or compressor) with oxygen (green hose) in a blender system to deliver a set FiO2. If set to a high enough flow, the patient cannot out-breathe this device, so the FiO2 is completely controlled. Again, high-flow means control, not just 100% FiO2 all the time.
Why does any of this matter?
Imagine you're treating a hypotensive patient with norepinephrine. You believe that you're infusing 30 mcg/min. However, in taking a closer look at the bag of norepinephrine, you realize that it's actually half the concentration that you thought it was (4mg in 250mL instead of 8mg in 250mL). Does this change things? Of course it does. You thought you were maxed out (if 30 mcg/min is your max), but now you realize you're only halfway there, and this treatment needs to be augmented before moving to another option or adding something else to the mix.
The same is true for oxygen delivery. If we believe that we're 'maxed out' and delivering 100% FiO2 to our patient, we might be tricked into thinking the current SPO2 or PO2 is the best we're going to get. However, is our oxygen actually more diluted with room air than we thought? Just like the medication above was more diluted with saline than we thought? We need to optimize flow, and/or bring in additional pressure, and/or try other maneuvers to increase oxygenation at that point.
Conclusion
Don't be tricked into thinking that you're delivering 100% FiO2 if you're really not. While we generally can't tell what our patient's inspiratory flow rate is without a ventilator, understanding the rise of inspiratory flow rates can trigger us to use more flow and/or pressure when we might not have believed it was necessary before. This knowledge is useful in mechanical ventilation as well - increasing flow rates increase synchrony with the ventilator because it meets the inspiratory flow demands of the patient. This concept will also help us not suffocate our patient - so I guess that's worth the 10 minutes you spent reading this blog.
Peer Review
Tyler Christifulli - FP-C
Sam does an excellent job describing not only the math and scientific reasoning of FiO2 delivery, but also gives excellent examples of how this impacts our decision making in the field. While not every patient needs 100% of their breath to be oxygen, there are specific times this is very important. For example, when pre-oxygenating a patient before intubation, the goal is to come as close as possible to 100% oxygen being delivered not to the mouth/nose - but to and through the alveoli, and into the pulmonary circulation. Sam shows a picture of a patient entraining air while on non-invasive mechanical support. It is important to note that the ability to entrain air will largely depend on if the mask is vented, and what device it is attached to.
For example, if connected to an oxygen regulated pressure device such as the Flow Safe, room air is able to be entrained. However, if connected to a mechanical ventilator, the only air the patient will receive will be regulated by the ventilator. This means a patient breathing at 60 lpm on 100% fiO2, will be using 60lpm of oxygen. For example, let's say I have 2000 PSI in my helicopter main oxygen. I know that aircraft that has an oxygen cylinder constant of 0.75, will only allow me 22.5 minutes of oxygen. The formula is shown below on our daily checklist.
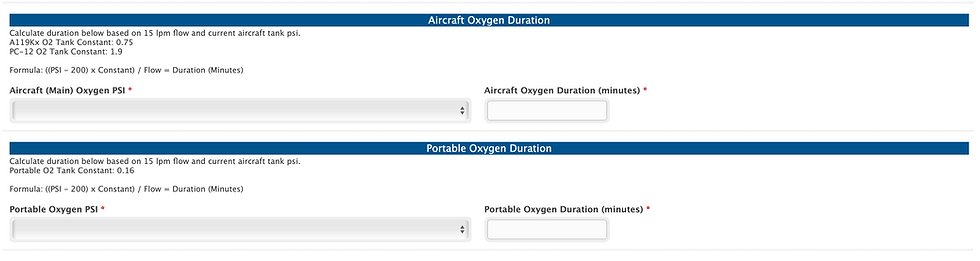
When we adopted the Sea-Long helmet CPAP device, it became salient for every clinician to understand everything mentioned in this blog. This helmet does not entrain room air and the flow delivered needs to meet the patient's demand. If demand is not met, the patient will quickly become anxious. Knowing when a hospital oxygen delivery strategy will not work in transport can prevent the dreaded "running out of oxygen in transport" nightmare.
If your oxygen delivery device and capabilities can not meet the patient's demand/condition, intubation and mechanical ventilation may be the best option for safe and effective transport.
References
Kacmarek, R., Stoller, J., Heuer, A., Chatburn, R. & Kallet, R. (2017). Egan's fundamentals of respiratory care. St. Louis, Missouri: Elsevier.
Markovitz GH, Colthurst J, Storer TW, Cooper CB. 2010 Apr;55(4):453-9. Effective inspired oxygen concentration measured via transtracheal and oral gas analysis. Respir Care.
Cairo, J. (2014). Mosby's respiratory care equipment. St. Louis, Mo: Elsevier.
Chang, D. (2014). Clinical application of mechanical ventilation. Clifton Park, New York: Delmar Cengage Learning.
Comments