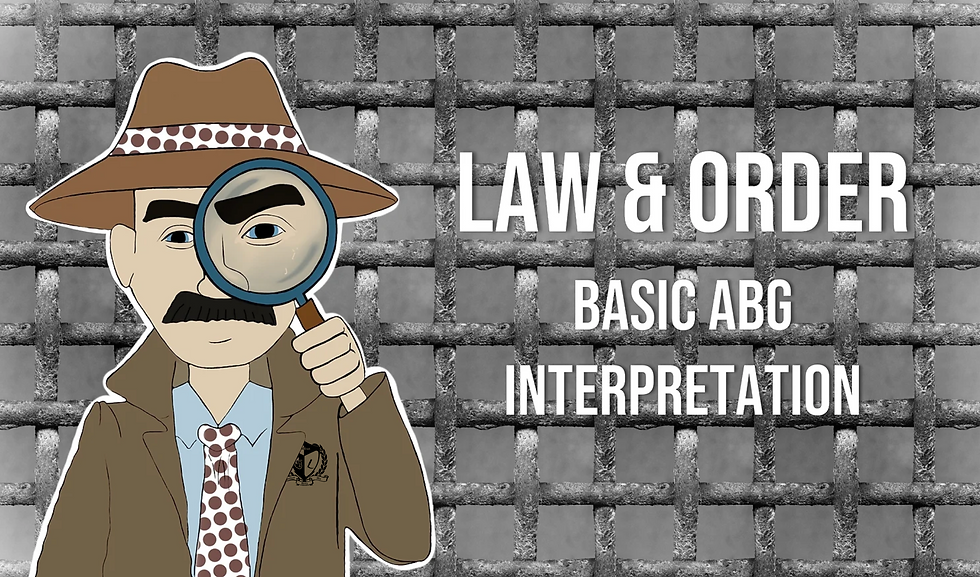
Arterial blood gases (ABG) can be pretty intimidating, especially for providers who are new to critical care medicine. The principles underlying ABGs are undoubtedly complex and involve a lot of chemical reactions – all of which are very important but likely overwhelming if you’re just beginning to learn ABG interpretation. This discussion is not intended to teach you everything there is to know about acid-base balance and ABGs, but rather to provide a functional base on which to build your understanding. So, we’ll leave out the fancy equations (minus one foundational chemical reaction) and frilly science and learn to interpret ABGs by putting to use those savvy detective skills you gained from alllll of those years spent watching low-budget detective shows between calls.
Basic Physiology
Cellular respiration - please keep reading, I promise I will not talk about the Krebs cycle - is at the core of all life-sustaining biologic processes. It produces energy for the body and can be completed with (aerobic) or without (anaerobic) oxygen. Aerobic cellular respiration offers the most efficient energy production, creating water and carbon dioxide (CO2) as byproducts. Anaerobic cellular respiration occurs when cellular oxygen consumption outweighs oxygen availability, produces less usable energy, and creates lactic acid as a byproduct.
Intrinsic balance of blood pH is managed largely by the balance of carbon dioxide and bicarbonate. Gas exchange in the alveoli passes off oxygen molecules from inspired air and excess CO2 molecules from venous blood. The respiratory system is driven by chemoreceptors in the brain, which are sensitive to fluctuations in CO2 concentration. Increased CO2 in the blood triggers increased minute volume in order to offload more CO2; conversely, low CO2 concentrations trigger hypoventilation to retain extra. Retained CO2 molecules combine with water to form carbonic acid. Carbonic acid dissociates (breaks apart) and becomes a hydrogen ion (driver of acidosis) and a bicarbonate ion (pH buffer). Bicarbonate is also monitored and produced by the kidneys as needed to balance hydrogen ions. In healthy patients, this mechanism maintains blood pH between 7.35 and 7.45; however, disruptions to normal physiology can overwhelm this balance and lead to acidosis or alkalosis.
There are four main types of acid-bace disturbances, which result from a variety of underlying pathologies. Regardless of the type of disturbance, the ultimate treatment is to treat the originating cause. Acid-base imbalances can be complicated by additional factors and at times, more than one etiology can concomitantly affect the patient. For the purposes of this discussion, we’ll stick to the four basic types:
o Respiratory acidosis results from inadequate alveolar ventilation leading to increased CO2 retention in the bloodstream. There are many underlying causes, such as stroke, central nervous system depression (opioid overdose, etc.), and muscular insufficiencies from conditions such as myasthenia gravis.
o Respiratory alkalosis results from hyperventilation secondary to some other pathology. Central causes of respiratory alkalosis include stroke, traumatic head injury, pain, anxiety, salicylates, and other toxins. Hypoxia may also drive respiratory alkalosis as the body attempts to increased oxygen intake via increased minute volume and inadvertently offloads too much CO2. Hypoxia-related causes include pneumonia and pulmonary embolism, among others.
o Metabolic acidosis has four primary causes: overproduction of acid (anaerobic cellular respiration -> lactic acid production), under-excretion of acid, ingestion of acid, and loss of bicarbonate through the renal (tubular acidosis) or GI system (diarrhea).
o Metabolic alkalosis can occur secondary to loss of hydrogen ions – without adequate hydrogen ions to bind and form carbonic acid, bicarbonate concentrations in the blood can increase beyond normal levels. Loss of hydrogen ions, and secondarily high bicarbonate levels, can occur due to vomiting (loss of stomach acid), intracellular ion shifts, renal ion loss, or hypovolemia.
Components of an ABG
pH is a measure of how acidic or basic a substance is. There are no units associated with pH. The scale runs from 0 to 14, with 0 being extremely acidic and 14 being extremely basic. In general chemistry, true neutral is 7. The normal pH range for human blood is slightly alkalotic, sitting between 7.35 – 7.45. Blood pH less than 7.35 is considered acidemia and pH above 7.45 is considered alkalemia. Even slight deviations outside of this range can result in significant physiologic disturbances.
pCO2 is the concentration of carbon dioxide circulating within the blood. The normal range for pCO2 is 35-45mmHg; for those familiar with ETCO2 use, this one is easy to remember. CO2 retention, or levels of pCO2 greater than 45mmHg, generally contribute to increased acidity – one possible exception to this is in the case of chronic restrictive lung pathologies like COPD or emphysema, where the patient’s body may have adjusted over time to a pCO2 greater than typical norms. Excessive CO2 offloading, reflected by pCO2 less than 35mmHg, generally contributes to alkalinity. In the event of a metabolic disturbance, the respiratory system may drive changes in pCO2 to maintain pH within the homeostatic range of 7.35 – 7.45.
pO2 is a measure of oxygen molecules within the blood and has a normal range of 80-100mmHg. While pO2 is important in understanding how effectively the patient is being oxygenated and may give insight into how other pathogies might interplay with the patient's metabolic disturbance, we won’t talk about it much here as it doesn’t play a heavy role in pH balance alone.
Finally, HCO3- is the measure of bicarbonate ions in the blood stream. The normal range for this varies slightly by source but is generally somewhere between 22-26mEq/L. Inadequate levels of bicarbonate may contribute to acidosis, while excessive bicarbonate ions may lead to alkalosis. Much like pCO2 is adjusted to compensate for metabolic disturbance, bicarbonate levels may fluctuate in the event of pH imbalance of respiratory origin.
Understanding ABGs
If you’ve received any education about acid-base balance and/or ABG interpretation in the past, you may have seen a chart with approximately 10,000 arrows that tells you whether you’re dealing with a respiratory acidosis, respiratory alkalosis, metabolic acidosis, or metabolic alkalosis. That chart never worked for me – way too much going on there - and while it might help you identify the type of imbalance, it doesn’t really offer us any functional understanding. If you’ve struggled to get a strong grasp of these
concepts in the past, hopefully this explanation helps you.
In a functional sense, CO2 can be considered both the acid component and the respiratory component of the ABG . Likewise, HCO3- can be considered both the basic component and the metabolic component of the ABG. In homeostasis, a perfect balance of acid and base exists. If there is too much acid or not enough base, the blood becomes acidic. If there’s not enough acid or too much base, the blood becomes basic. We can use this knowledge to identify both the origin of the disturbance and whether it is compensated or uncompensated. If this doesn’t make sense now, hopefully it will in a few minutes.
Let’s apply this to a few examples in a stepwise process. This is where you get to put your detective hat on. To solve the case, we just have to answer three questions.
1. What kind of crime has been committed? (acidosis or alkalosis)
2. Who is the culprit? (respiratory or metabolic)
3. Did someone try to cover up the crime? (compensated vs uncompensated)
Example 1
1. To figure out which time of crime - or disturbance - has occured, first look at the pH. If it’s less than 7.35, you’ve got an acidosis on your hands. If it’s greater than 7.45, it’s an alkalosis. See, this is easy.
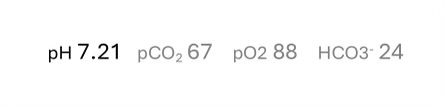
Because the pH is less than 7.35, we can confidently call this an acidosis.
2. Now, we look for the culprit – which component, pCO2 or HCO3- is consistent with an acidosis? We'll look at the pCO2 next.
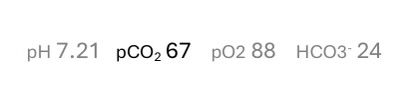
This pCO2 is above the normal range of 35-45. We have TOO MUCH of the acid component, which does explain the low pH (acidosis). It seems the pCO2 (respiratory component) is the culprit - looks like we have a respiratory acidosis on our hands.
3. Finally, since we know the respiratory system was the culprit, we need to figure out if bicarb was in on it too. That is – did bicarb change to try to compensate for the disturbance? Let’s evaluate the HCO3-.
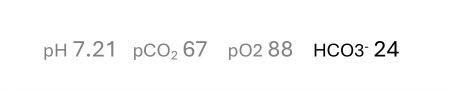
The HCO3- is within the normal range. It has not yet increased to compensate for the excess acid. Therefore, this is an uncompensated respiratory acidosis.
Example 2
Here’s another example. We’ll use the same pH of 7.21 – another acidosis.
This time, however, the pCO2 is normal and does not correlate with an acidosis. Seems like the respiratory system can be cleared from this crime… at least for now. Once again, we’ll move onto the bicarb.
At 9, the bicarb is way below normal range. Since we know that inadequate amounts of bicarb can contribute to acidosis, it does explain the low pH. And – since we recognize bicarb as our metabolic component – we can call this a metabolic acidosis. To determine compensated versus uncompensated, all we need to do is hop back over to the pCO2. Since it was within normal range at 37mmHg, this is an uncompensated metabolic acidosis.
A few more rapid-fire examples
Breaking this ABG down the same way as the others, we first notice that the pH is greater than 7.45 – an alkalosis. But woah! The pCO2 is high, which is actually consistent with an acidosis, so we know that isn’t the cause of our imbalance. Ignore that for now – we’re still looking for the culprit.
At 32, the bicarb is overabundant and is consistent with the alkalosis reflected in the pH. This tells us we have an underlying metabolic alkalosis. Now the increased pCO2 makes sense – the body is retaining additional CO2 to compensate for the high bicarb. The respiratory system is trying to cover up the crime but didn’t quite finish the job, since the pH is still outside of the normal range. This is a partially compensated metabolic alkalosis. Okay, next.
This pH is normal – so there’s no underlying disturbance, right? Not so fast, detective.
Following the same stepwise process, you’ll notice that the pCO2 high – we have extra acid and would expect to see an acidosis. The bicarb is elevated as well, so we also have extra base. Since both of these components are outside of their normal ranges, but the pH is normal, we know this is a fully compensated ~something.~
Because the pH is on the low end of normal (leaning towards the acidotic side - you can use 7.40 as the midpoint when dealing with gases like this), it’s most likely that the patient's underlying disturbance is an acidosis rather than an alkalosis. The elevated pCO2 is the value consistent with an acidosis (the culprit), and the elevated bicarb in turn explains the balanced pH (the coverup); so, this is a fully compensated respiratory acidosis. They almost got away with it that time.
Closing Thoughts
Hopefully this silly exercise helped you make sense of ABGs and gain a basic understanding of acid-base balance. Again - I want to stress that this only scratches the surface of these topics. Blood gases should be used as an adjunct to patient care, not sole drivers of decision-making. While blood gases can be useful tools, it's critical that providers use good judgement and consider the overall clinical picture before making any treatment decisions.
References
Castro, Danny, and Michael Keenaghan. “Arterial Blood Gas.” National Library of Medicine, StatPearls Publishing, 8 Jan. 2024, www.ncbi.nlm.nih.gov/books/NBK536919/. Accessed 28 July 2024.
Hopkins, Erin, et al. “Physiology, Acid Base Balance.” National Library of Medicine, StatPearls Publishing, 12 Sept. 2022, www.ncbi.nlm.nih.gov/books/NBK507807/. Accessed 28 July 2024.
Burger M, Schaller DJ. Metabolic Acidosis. www.ncbi.nlm.nih.gov. Published July 17, 2023. https://www.ncbi.nlm.nih.gov/books/NBK482146/?report=reade
Brinkman JE, Sharma S. Physiology, Metabolic Alkalosis. PubMed. Published July 23, 2023. Accessed August 8, 2024. https://www.ncbi.nlm.nih.gov/books/NBK482291/
Brinkman JE, Sharma S. Physiology, respiratory alkalosis. Nih.gov. Published July 24, 2023. Accessed August 8, 2024. https://www.ncbi.nlm.nih.gov/books/NBK482117/
Patel S, Sharma S. Respiratory Acidosis. National Library of Medicine. Published June 12, 2023. Accessed August 8, 2024. https://www.ncbi.nlm.nih.gov/books/NBK482430/
Comments