Brain Bleeds and Heart Blocks
- Sam Ireland
- Dec 27, 2020
- 7 min read
A patient falls off of a ladder from a significant height. You arrive on the scene to find that they landed on their back, on concrete. A twisted left lower extremity reveals a femur fracture, and the patient has been exacerbating the injury due to their combativeness. In fact, the left thigh is growing in size by the second. You now realize you’re working with multi-system trauma. The patient has one or more major bleeds, as well as an obvious head injury from the fall (we know this from the combative nature of the patient and from observing external trauma to the back of the head). How would you expect this patient's vital signs to present?
You would likely expect to see:
Hypertension (some sources substitute widening pulse pressure)
Bradycardia
Irregular breathing patterns
Known as? Cushing’s Reflex! The three stages above generally present in the order listed. It starts with hypertension, followed by bradycardia and then irregular breathing patterns that may include apnea. This is how we’ve classically been taught that a patient with increased intracranial pressure will present. In short, the body recognizes that the brain isn’t getting adequate perfusion, so the body cranks up the pressure. But, where do the bradycardia and irregular breathing patterns come from? It all has to do with the...
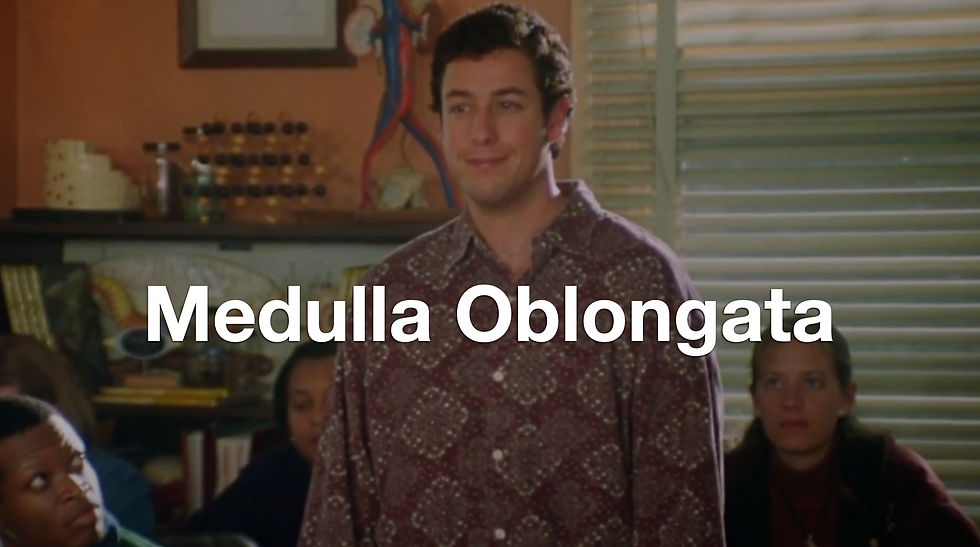
The Medulla Oblongata is part of the brainstem, and it contains centers that regulate our heart rate, blood pressure, and breathing. (The Pons also play a key role in respiratory patterns and is located just above the Medulla.) When the Medulla receives signals from the body, it sends messages back for the body to make adjustments. How are these messages received by the Medulla and Pons and sent back to the body?
IX, X, X
The key nerves that carry these messages up to the brain are the glossopharyngeal (IX) and Vagus (X) nerves. Then, a different branch of the Vagus (X) nerve carries the messages back down to the heart. Nerves IX and X, are baroreceptor nerves that sense pressure in the aortic arch and carotid arteries then communicate that pressure reading up to the medulla (Lau, 2016). As a side note, the respiratory centers receive messages through the same nerves (afferent IX and X) to the Medulla and Pons, except their messages get transferred back to the body via sympathetic nerves such as the efferent phrenic nerve through the spinal cord. Remember how 'C3, 4, and 5 keep the diaphragm alive'? That's the phrenic nerve. Also, the respiratory nerves are chemoreceptors, sensing oxygen and carbon dioxide instead of pressure. Whew. That's some heavy stuff. Since we are focusing on the heart's reaction in this blog, let's put the heart rate control into picture form so we can visualize all these messages going back and forth!
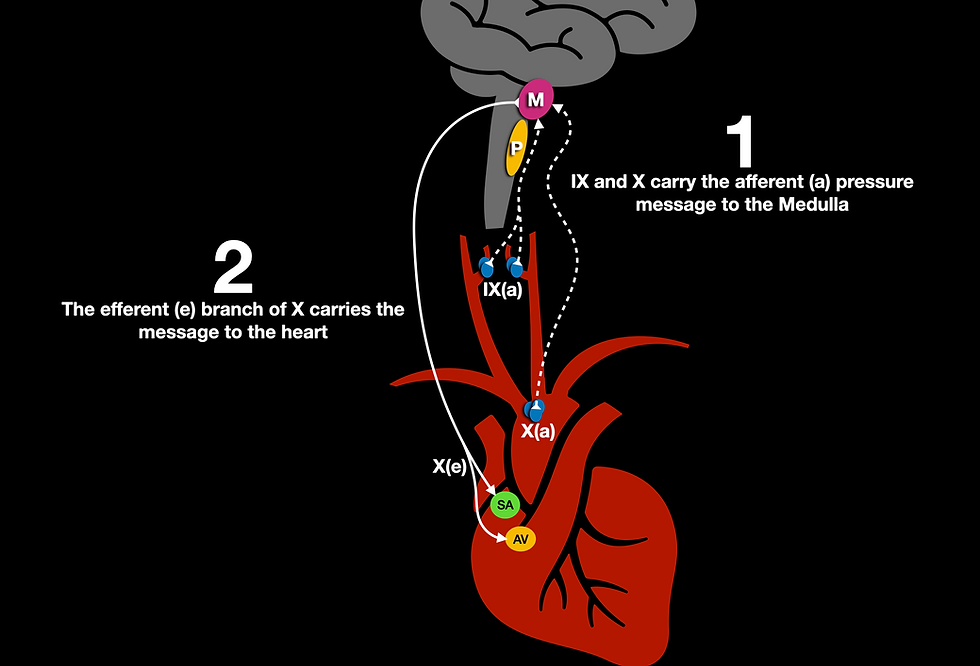
This whole process of conveying a message to the brainstem about pressure is known, as I mentioned earlier, as Cushing's Reflex. Cushing's reflex is a pathological version of a reflex known as the baroreceptor reflex. "Baro" is a prefix meaning 'weight' or 'pressure.'
The baroreceptors in the aortic arch and the carotid arteries are pretty simple to understand - they convey a message to the brainstem about the current pressure in the arteries. The brain then interprets those messages and sends messages back to the body about how to use this information.
A Body in Disagreement?
Here's something I really struggled to understand about Cushing's reflex... If the pressure becomes too high and the body tries to lower the pressure, why doesn't the pressure in a head bleed patient ever seem to come down? The pulse rate gets low, but the blood pressure is still sky-high. Doesn't it seem like the body is disagreeing with itself? Why does this occur? To understand this mechanism, we have to add sympathetic nerves to the mix.
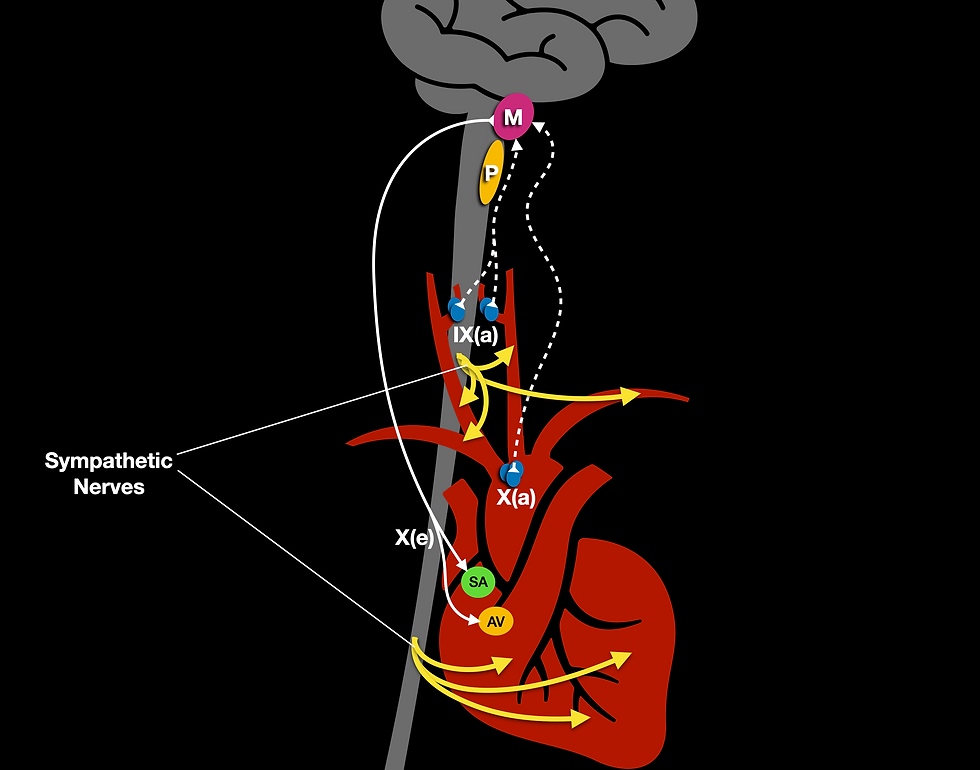
The Vagus (X) nerve may have the SA and AV node on lockdown, but guess who's majorly pulling the strings in the ventricles and arteries?
The sympathetic nervous system!
This fight or flight portion of the nervous system is completely opposite of its parasympathetic (feed/breed) counterpart, and it has zero chill. While the Vagus nerve is telling the SA and AV node to slow down, the sympathetic nerves are still telling the ventricles to increase inotropy (squeezing force). Also, the sympathetic nervous system has some vasoconstriction going on, which increases the SVR (systemic vascular resistance). Through this dichotomy of territory (areas in influence), the parasympathetic system wins some, and the sympathetic system wins some. This is how we get bradycardia, yet still, have hypertension. If we add the abnormal respiratory response from above, we have all the ingredients for our Cushing's reflex (Dinallo, 2020).
Unilingual
The Baroreceptors are unilingual - they only speak the language of pressure. If the baroreceptors don't see an increase in pressure, they won't be triggered (they won't send a message to the brain stem). How does this relate to our patient at the outset? Let's go back and look at our patient who fell from the ladder.
This patient had multi-system trauma. He had a head injury, but he was also exsanguinating. When there is a leak in the system (such as a massive pelvic or femoral bleed), how can the pressure ever build up? If the pressure never builds up to trigger the baroreceptors, we won't see the bradycardia from Cushing's reflex - we will likely see tachycardia instead (tachycardia is also usually present in the very acute phase prior to the bradycardia). Why does this matter?
Your patient is still very likely experiencing increased ICP, but they are not presenting with the classic Cushing's triad. This may lead to delayed care and possibly even a missed diagnosis.
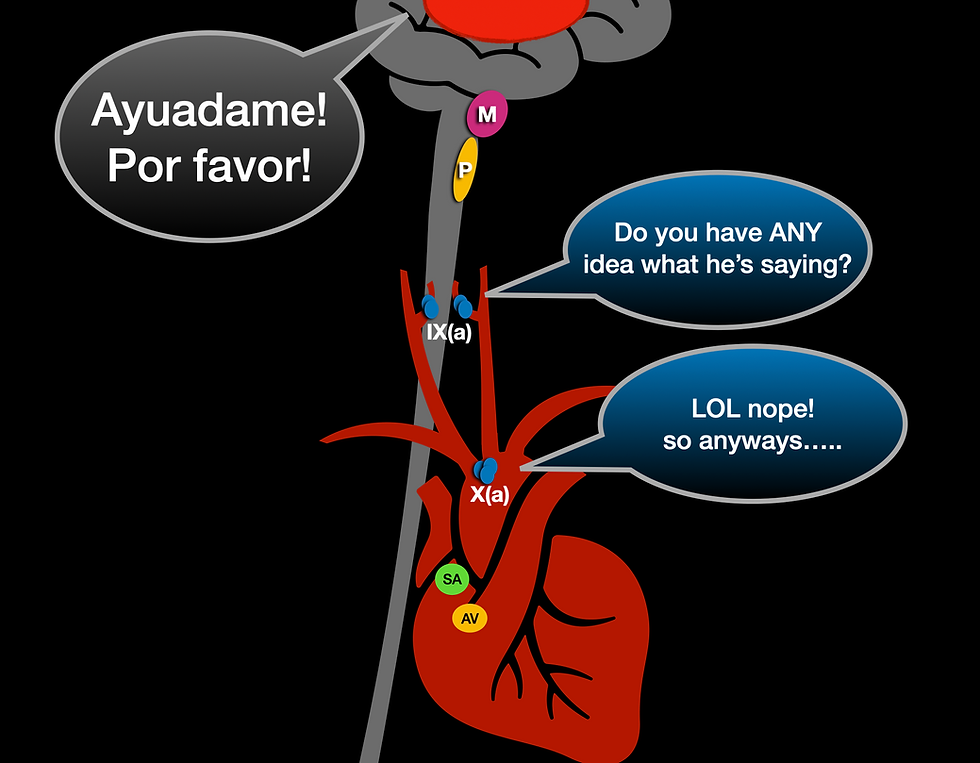
Reversal
If the patient is able to maintain hypertension (not exsanguinating), the stimulation from the Vagus nerve could be so strong that it causes more pathological versions of bradycardia (Park et al, 2019). This could result in high degree AV blocks or SA nodal exit blocks as well. What causes these conduction delays? The very strong vagal tone from the parasympathetic system stimulates the M2 Muscarinic Acetylcholine Receptor, resulting in slowed conduction.
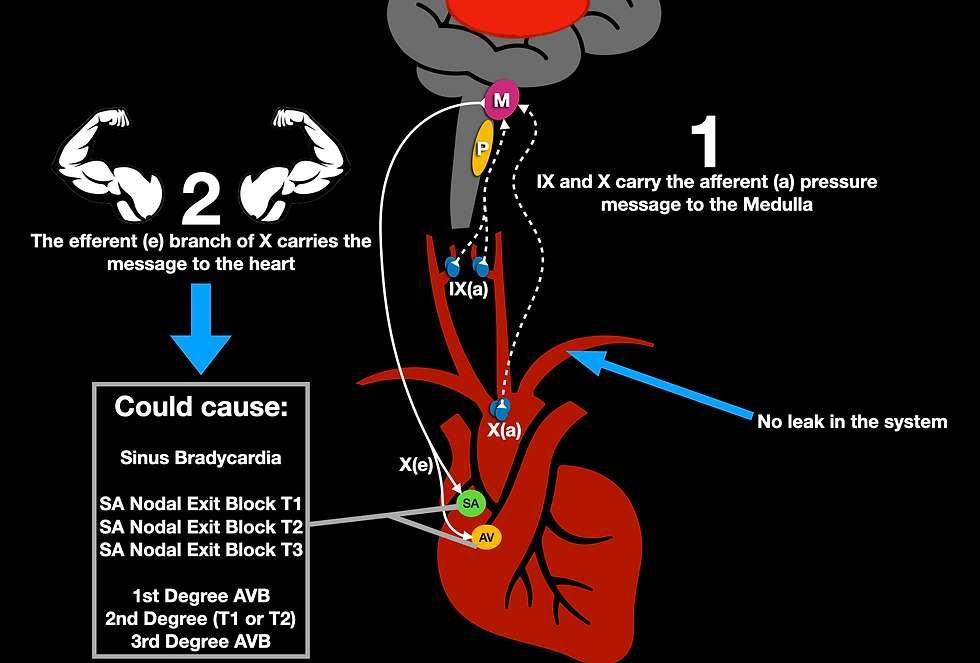
Any cause of increased vagal stimulation could cause these blocks if it's strong enough. By the way, if your instructor failed you and never told you about SA nodal exit blocks, you can check them out here. Alright, let's wrap this up.
What's the point of bringing up that these conduction blocks are Vagus nerve mediated? Because we often assume that the heart will not respond to Atropine if we have a high degree block. And, this is usually true. If there is a high degree AV block, and the atria and ventricle are not communicating, Atropine will usually only increase the rate of the atria, which again, will not be communicated to the ventricle since the AV node is not sending the message through (Knabben, 2020). However, if the cause of the AV block is purely parasympathetic in nature, giving a parasympatholytic medication (Atropine) to blunt acetylcholine may actually fix the conduction block. So, that recommendation from the AHA to try Atropine despite the level of AV block might not be so silly after all (1mg is now the 2020 AHA recommendation from the ACLS update).
Conclusion
Clearly, there is a lot to know about this rather simple reflex. The presentation of brain bleeds and heart blocks are both going to depend on how strong that parasympathetic tone is when it's transferred to the SA and AV nodes. If there is a leak in the system, we may never reach high enough pressures in the aortic arch or carotid arteries to cause any kind of bradycardia. However, if there is no leak in the system and pressure builds, our parasympathetic nervous system may have a heyday with our heart's conduction system. And, if our pathologic bradycardia is mediated by parasympathetic activity, lysing the parasympathetic nervous system with Atropine may actually reverse that blockade. If bradycardia is negatively impacting cerebral perfusion pressure (cardiac output or the ability to maintain adequate blood pressure), it may warrant treatment since hypotension is a serious contributor to mortality in increased ICP - consult your local guidelines or medical control.
Peer Review
Tyler Christifulli, FP-C
FOAMfrat Peer Review:
Shortly after our last podcast "TBI+Multisystem Trauma w/ Jake Good," I received an email from a clinician who stated:
"Hey man, I was just listening to your podcast 108, the one on TBI's + Multisystem trauma. I 100% agree that looking for Cushing's triad to clue you into increased ICP, can be misleading."
This blog is a nice compliment to that podcast because it highlights very clearly, why this reflex is a dependent variable. As I was reading this, I kept thinking about an intra-aortic balloon pump. When the balloon inflates, the pressures between the closed aortic valve and the distal tip of the balloon can skyrocket during diastole. While this is only for a second, I do wonder what the time+pressure threshold is for this response. Could a tachycardic patient on a balloon create a mean baroreceptor pressure high enough to cause reflexive bradycardia?
Excellent and thought-provoking write up.
Brian King
FOAMfrat Peer Review:
This is the type of content that we need to see more of in paramedic textbooks. I took a gander at a recently published paramedic textbook and 8 whole sentences were devoted to Cushing's reflex. It is imperative that prehospital clinicians not only know what to look for but also have a fundamental understanding of the specific pathology.
While Cushing's reflex is most often associated with intracranial hypertension in the setting of a TBI it is also important to remember that this reflex can be seen in any condition in which the ICP is increased such as cerebral edema, tumor, hemorrhagic stroke, etc.
It is important to have a high index of suspicion for increased ICP prior to a patient developing bradycardia & hypertension as they are often a late finding of intracranial hypertension and carry a high mortality. In the setting of isolated TBI it may be worth considering the value of tachycardia and hypertension as an early sign of intracranial hypertension prior to seeing late sings such as Cushing's reflex.
Excellent write up!
References
Park, J; Tavares, M; Kanemo, P; Weissman, A. 2019.
HIGH-DEGREE ATRIOVENTRICULAR BLOCK WITH SUPERIMPOSED SINOATRIAL NODE EXIT BLOCK SECONDARY TO INCREASED INTRACRANIAL PRESSURE.
Dinallo S, Waseem M. Cushing Reflex. [Updated 2020 May 24]. In: StatPearls [Internet]. Treasure Island (FL): StatPearls Publishing; 2020 Jan-. Available from: https://www.ncbi.nlm.nih.gov/books/NBK549801/
Knabben V, Chhabra L, Slane M. Third-Degree Atrioventricular Block. [Updated 2020 Aug 8]. In: StatPearls [Internet]. Treasure Island (FL): StatPearls Publishing; 2020 Jan-. Available from: https://www.ncbi.nlm.nih.gov/books/NBK545199/
Lau, E. O., Lo, C. Y., Yao, Y., Mak, A. F., Jiang, L., Huang, Y., & Yao, X. (2016). Aortic Baroreceptors Display Higher Mechanosensitivity than Carotid Baroreceptors. Frontiers in physiology, 7, 384. https://doi.org/10.3389/fphys.2016.00384
Comments